Neutron Beam More Accurate Probe Than X-ray, Could Also Be Used to Find Physics’ ‘Fifth’ Forces
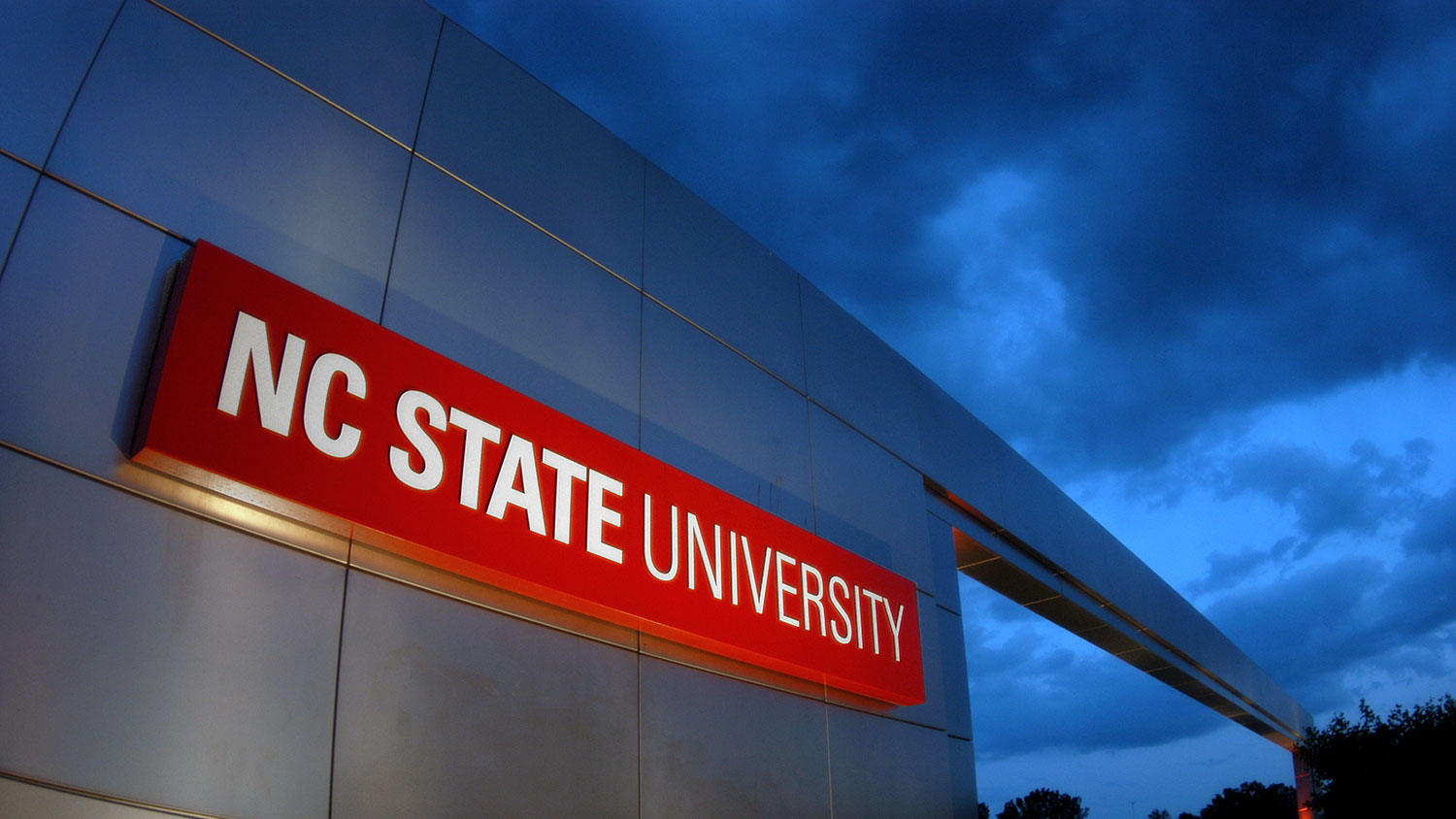
For Immediate Release
An international group of researchers performed pendellösung interferometry on silicon using a neutron beam, providing both the highest precision measurements with this technique to date, and a “snapshot” of the properties of both the silicon crystal and the neutron itself. The neutron beam’s unique properties also make it suitable for potentially detecting the presence of a “fifth force,” elusive forces connected to some of the deepest mysteries of physics, such as the existence of dark energy or extra spatial dimensions.
Pendellösung interferometry is an optical technique which has been used to determine crystal material properties – particularly of silicon – such as its mechanical and thermal properties and how the forces holding it together (the atoms within it) are arranged.
“Even though silicon is ubiquitous, we are still learning about its most basic properties,” says Albert Young, professor of physics at North Carolina State University and co-author of the research. “The neutron, because it has no charge, is excellent to use as a probe because it doesn’t interact strongly with electrons inside the material. X-rays have some drawbacks when measuring atomic forces within a material due to their interaction with electrons.”
The research team used a neutron beam at the National Institute of Standards and Technology’s (NIST) Center for Neutron Research to perform pendellösung interferometry on a perfect silicon crystal. The team was led by Benjamin Heacock while he was a graduate student at NC State, and worked closely with NIST instrument scientist, Michael Huber.
Although crystal structure studies are typically performed with X-rays, a neutron beam is orders of magnitude less sensitive to atomic electron density than X-rays, which makes it an ideal probe of the vibrational properties of the silicon crystal.
A perfect silicon crystal is one in which the sheets of atoms in each interior plane are identical, and their spacing and orientation repeat throughout the entire crystal. Neutrons interact with these planes as a quantum wave, and when the planes are aligned as in a perfect crystal, the neutron beam can reflect repeatedly from the crystal planes. Each reflection bounces neutrons off of the atoms in the crystal, and the reflecting waves encode these interactions. When the neutron wave finally escapes from the crystal, the bounce history provides data on how the atomic forces are arranged and how they influence the neutron.
The silicon crystal was oriented very precisely to pick out a particular reflection condition. The crystal was then rotated around a perpendicular axis to produce the pattern of waves which gave the team data about how the atomic forces inside the crystal were arranged.
Prior to this work, pendellösung had been performed on only one alignment of the beam with respect to the crystal planes (known as a Bragg condition). In this work two new Bragg conditions were measured with neutrons and the precision was improved by about a factor of four.
“Unlike previous pendellösung measurements, our technique gives you the properties of and forces within the crystal super precisely – including the neutron charge radius and short-range forces – which add to our understanding of not just silicon, but of neutrons themselves,” Young says.
Short-range forces refer to as yet undiscovered forces that physicists have theorized exist but which haven’t been observed, which can be connected to dark energy, or new theories of gravity with extra spatial dimensions. Young hopes that probing short-range forces could give scientists insights into these larger questions. The work also provides new tools and a new community of scientists who can address these questions using small scale experiments.
“The great thing about this work is not only the precision – we can hone in on specific observables in the crystal – but also that we can do it with a tabletop experiment, not a large collider,” Young says. “Making these small-scale, precise measurements could make progress on some of the most challenging questions for fundamental physics.”
The research appears in Science and was supported by the U.S. Department of Energy (grants 89243019SSC000025 and DE-FG02-97ER41042), the National Science Foundation (grant PHY-1307426), the Natural Sciences and Engineering Council of Canada Discovery program, and the Canada First Research Excellence Fund. Heacock is first author. Co-investigators included researchers from NIST; Nagoya University, Japan; Tulane University; the University of Waterloo, Canada; RIKEN center for advanced photonics, Hirosawa, Japan; Triangle Universities Nuclear Laboratory, NC; and the High Energy Accelerator Research Organization, Tsukuba, Japan. Paul Huffman, professor of physics at NC State, also contributed to the work.
-peake-
Note to editors: An abstract follows.
“Pendellössung Interferometry Probes the Neutron Charge Radius, Lattice Dynamics, and Fifth Forces”
Authors: Benjamin Heacock, Robert Valdillez, Albert Young, North Carolina State University; Albert Henins, Michael Huber, NIST; Takuhiro Fujie, Katsuya Hirota, Masaaki Kitaguchi, Hirohiko Shimizu, Nagoya University, Japan; Takuya Hosobata, Masahiro Takeda, Yutaka Yamagata; RIKEN Center for Advanced Photonics, Saitama, Japan; Robert Haun, Tulane University; Dmitry Pushin, University of Waterloo, Ontario, Canada
Published: Sept, 10, 2021 in Science
Abstract:
Structure factors describe how incident radiation is scattered from materials such as silicon and germanium and characterize the physical interaction between the material and scattered particles. We use neutron pendellosung interferometry to make precision measurements of the (220) and (400) neutronsilicon structure factors, and achieve a factor of four improvement in the (111) structure factor uncertainty. These data provide measurements of the silicon Debye-Waller factor at room temperature and the mean square neutron charge radius. Combined with existing measurements of the Debye-Waller factor and charge radius, the measured structure factors also improve constraints on the strength of a Yukawa-modification to gravity by an order of magnitude over the 20 pm to 10 nm length scale range.
- Categories: