New Technique Improves Directed Evolution of Microorganisms
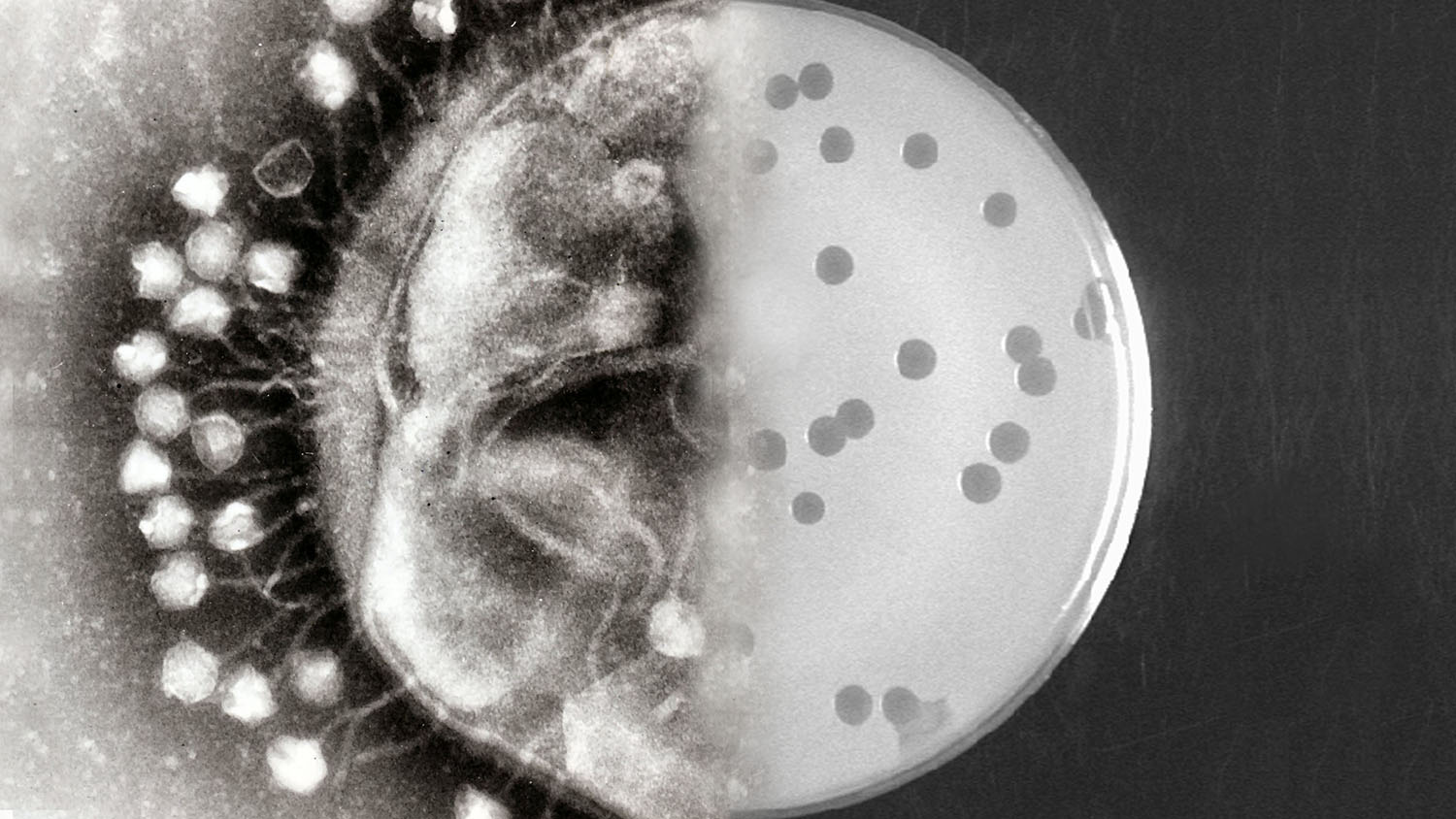
For Immediate Release
Researchers at North Carolina State University have developed and demonstrated a new technique for controlling directed evolution in bacteria. The technique can be used to better engineer microorganisms for use in biopharmaceutical and chemical manufacturing.
“Our technique allows users to modify 10 times more genes than previous techniques,” says Nathan Crook, corresponding author of a paper on the work and an assistant professor of chemical and biomolecular engineering at NC State. “Previous techniques for directed evolution allowed you to engineer up to three genes at a time. Our approach allows you to tweak up to 30 genes at a time. In addition, our approach is more amenable to automation than previous techniques, making the process significantly less difficult and time-consuming.”
The new technique, called Inducible Directed Evolution (IDE), differs from established directed evolution because it makes use of inducible lysogenic bacteriophages. Lysogenic bacteriophages are viruses that are capable of residing inside a host bacterium for an extended period of time before reproducing and destroying the cell. Specifically, the researchers used inducible lysogenic bacteriophages, which won’t reproduce and destroy the cell until receiving a specific signal. In this case, that signal is the introduction of a specific monosaccharide, or simple sugar.
“Other bacteriophages begin reproducing as soon as possible after being introduced into a cell,” Crook says. “If you’re using those bacteriophages to introduce engineered DNA into the cell, this means you have very little time for those engineered mutations to cause an observable change in the cell’s behavior or in the products that the cell is producing.
“By using inducible lysogenic bacteriophages, we can introduce a piece of engineered DNA into a cell and prevent the bacteriophage from reproducing right away. We can give the mutated DNA days or weeks to do its job before triggering the bacteriophage to reproduce. That gives us significantly more time for the engineered mutations to make changes in the cell’s behavior or to make the cell churn out whatever products we’re trying to create.”
To demonstrate IDE’s effectiveness, the researchers modified a strain of E. coli. Specifically, the researchers engineered the bacteria to efficiently consume a source of carbon that E. coli normally has trouble breaking down.
“Our demonstration shows that IDE is capable of making finely tuned changes to a bacterium,” Crook says. “And we chose E. coli because it is already widely used by industry to create biopharmaceuticals and other chemicals. What’s more, we think E. coli holds promise as a designer probiotic, and we think IDE could help fulfill that promise.”
The paper, “Inducible Directed Evolution of Complex Phenotypes in Bacteria,” is published in the journal Nucleic Acids Research. First author of the paper is Ibrahim Al’Abri, a Ph.D. student at NC State. The paper was co-authored by Daniel Haller, an undergraduate at NC State; and Zidan Li, a Ph.D. student at NC State.
-shipman-
Note to Editors: The study abstract follows.
“Inducible Directed Evolution of Complex Phenotypes in Bacteria”
Authors: Ibrahim S. Al’Abri, Daniel J. Haller, Zidan Li and Nathan Crook, North Carolina State University
Published: Feb. 12, Nucleic Acids Research
DOI: 10.1093/nar/gkac094
Abstract: Directed evolution is a powerful method for engineering biology in the absence of detailed sequence-function relationships. To enable directed evolution of complex phenotypes encoded by multigene pathways, we require large library sizes for DNA sequences >5-10kb in length, elimination of genomic hitchhiker mutations, and decoupling of diversification and screening steps. To meet these challenges, we developed Inducible Directed Evolution (IDE), which uses a temperate bacteriophage to package large plasmids and transfer them to naive cells after intracellular mutagenesis. To demonstrate IDE, we evolved a 5-gene pathway from Bacillus licheniformis that accelerates tagatose catabolism in Escherichia coli, resulting in clones with 65% shorter lag times during growth on tagatose after only two rounds of evolution. Next, we evolved a 15.4 kbp, 10-gene pathway from Bifidobacterium Breve UC2003 that aids E. coli’s utilization of melezitose. After three rounds of IDE, we isolated pathways that both reduced lag time by more than 2-fold and enabled 150% higher final optical density. Taken together, this work enhances the capacity for and demonstrates the utility of a whole pathway directed evolution approach in E. coli.