Researchers Set New Bar for Water-Splitting, CO2-Splitting Techniques
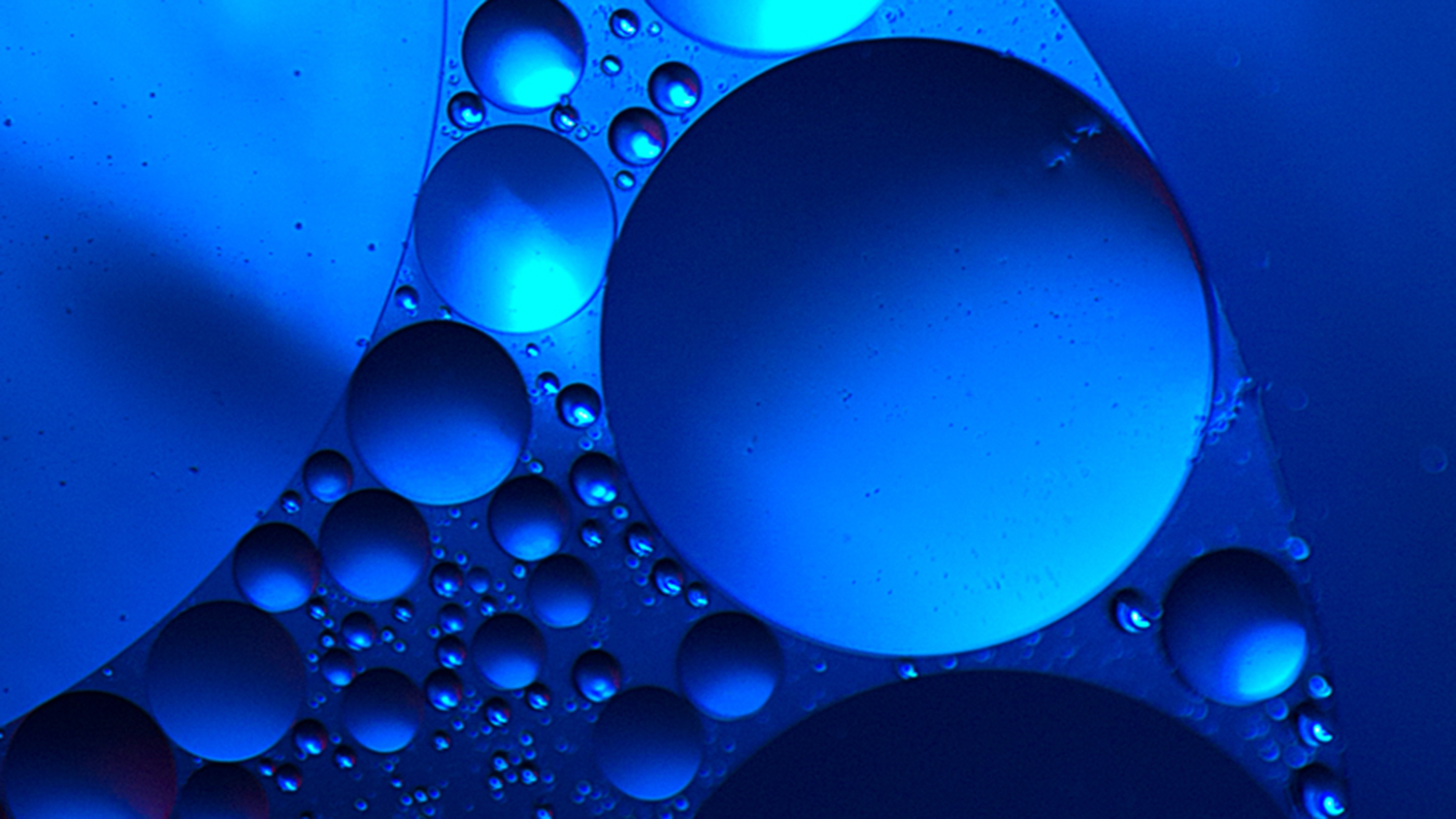
For Immediate Release
Researchers from North Carolina State University have significantly boosted the efficiency of two techniques, for splitting water to create hydrogen gas and splitting carbon dioxide (CO2) to create carbon monoxide (CO). The products are valuable feedstock for clean energy and chemical manufacturing applications.
The water-splitting process successfully converts 90 percent of water into hydrogen gas, while the CO2-splitting process converts more than 98 percent of the CO2 into CO. In addition, the process also uses the resulting oxygen to convert methane into syngas, which is itself a feedstock used to make fuels and other products.
“These advances are made possible by materials that we specifically designed to have the desired thermodynamic properties for each process,” says Fanxing Li, an associate professor of chemical and biomolecular engineering at NC State who is corresponding author of two papers on the work. “These properties had not been reported before unless you used rare earth materials.”
For the CO2-splitting process, researchers developed a nanocomposite of strontium ferrite dispersed in a chemically inert matrix of calcium oxide or manganese oxide. As CO2 is run over a packed bed of particles composed of the nanocomposite, the nanocomposite material splits the CO2 and captures one of the oxygen atoms. This reduces the CO2, leaving only CO behind.
“Previous CO2 conversion techniques have not been very efficient, converting well below 90 percent of the CO2 into CO,” Li says. “We reached conversion rates as high as 99 percent.
“And CO is valuable because it can be used to make a variety of chemical products, including everything from polymers to acetic acid,” Li says.
Meanwhile, the oxygen captured during the CO2-splitting process is combined with methane and converted into syngas using solar energy.
For the water-splitting process, researchers created iron-doped barium manganese oxide particles. Other than the difference in materials, the process is remarkably similar. As water – in the form of steam – is run over a bed of the particles, the iron-doped barium manganese oxide splits the water molecules and captures the oxygen atoms. This leaves behind pure hydrogen gas.
“Our conversion here is 90 percent, which compares very favorably to other techniques – which are often in the 10-20 percent range,” says Vasudev Haribal, a Ph.D. student at NC State and lead author of the paper on the water-splitting work.
The oxygen captured during the water-splitting process is used to make syngas, using the same technique used in the CO2-splitting process.
“We think both of these materials and processes represent significant steps forward,” Li says. “They use relatively inexpensive materials to efficiently extract valuable feedstock from resources that are either readily available (in the case of water) or are actually greenhouse gases (in the cases of CO2 and methane).
“We are now working on developing materials that are even more efficient,” Li says. “And we’re open to working with outside groups who are interested in scaling these processes up for manufacturing.”
The CO2-splitting paper, “Perovskite Nanocomposites as Effective CO2-Splitting Agents in a Cyclic Redox Scheme,” is published in the journal Science Advances. Lead author of the paper is Junshe Zhang, a former postdoctoral researcher at NC State who is now at Xi’an Jiaotong University. The paper was co-authored by Haribal. The work was done with support from the National Science Foundation, under grants CBET-1254351 and CBET-1510900, and the Kenan Institute at NC State.
The water-splitting paper, “Iron-Doped BaMnO3 for Hybrid Water Splitting and Syngas Generation,” is published in the journal ChemSusChem. The paper was co-first authored by Feng He, a former Ph.D. student at NC State, and Amit Mishra, a Ph.D. student at NC State. The work was done with support from NSF, under grant CBET-1254351, and the Kenan Institute at NC State.
-shipman-
Note to Editors: The study abstracts follow.
“Perovskite Nanocomposites as Effective CO2-Splitting Agents in a Cyclic Redox Scheme”
Authors: J.S. Zhang, North Carolina State University and Xi’an Jiaotong University; V. Haribal and F. Li, North Carolina State University
Published: Aug. 30, Science Advances
DOI: 10.1126/sciadv.1701184
Abstract: We report iron-containing mixed-oxide nanocomposites as highly effective redox materials for thermochemical CO2-splitting and methane partial oxidation in a cyclic redox scheme, where methane was introduced as an oxygen “sink” to promote the reduction of the redox materials followed by re-oxidation through CO2 splitting. Up to 96% syngas selectivity in the methane partial oxidation step and close to complete conversion of CO2 to CO in the CO2-splitting step were achieved at 900 – 980 °C with good redox stability. The productivity and production rate of CO in the CO2-splitting step were about 7 times higher than those in state-of-the-art solarthermal CO2 splitting processes, which are carried out at significantly higher temperatures. The proposed approach can potentially be applied for acetic acid synthesis with up to 84% reduction in CO2 emission when compared to state-of-the-art processes.
“Iron-Doped BaMnO3 for Hybrid Water Splitting and Syngas Generation”
Authors: Vasudev Pralhad Haribal, Feng He, Amit Mishra and Fanxing Li, North Carolina State University
Published: Aug. 7, ChemSusChem
DOI: 10.1002/cssc.201700699
Abstract: A rationalized strategy to optimize transition-metal-oxide-based redox catalysts for water splitting and syngas generation through a hybrid solar-redox process is proposed and validated. Monometallic transition metal oxides do not possess desirable properties for water splitting; however, density functional theory calculations indicate that the redox properties of perovskite-structured BaMnxFe1-xO3-δ can be varied by changing the B-site cation compositions. Specifically, BaMn0.5Fe0.5O3-δ is projected to be suitable for the hybrid solar-redox process. Experimental studies confirm such predictions, demonstrating 90% steam-to-hydrogen conversion in water splitting and over 90% syngas yield in the methane partial-oxidation step after repeated redox cycles. Compared to state-of-the-art solar-thermal watersplitting catalysts, the rationally designed redox catalyst reported is capable of splitting water at a significantly lower temperature and with ten-fold increase in steam-to-hydrogen conversion. Process simulations indicate the potential to operate the hybrid solar-redox process at a higher efficiency than state-ofthe-art hydrogen and liquid-fuel production processes with 70% lower CO2 emissions for hydrogen production.
- Categories: