Innovations of Ultrasound
By harnessing sound waves, scientists at NC State’s College of Engineering are pushing the boundaries of ultrasound technology and revolutionizing the field of medicine.
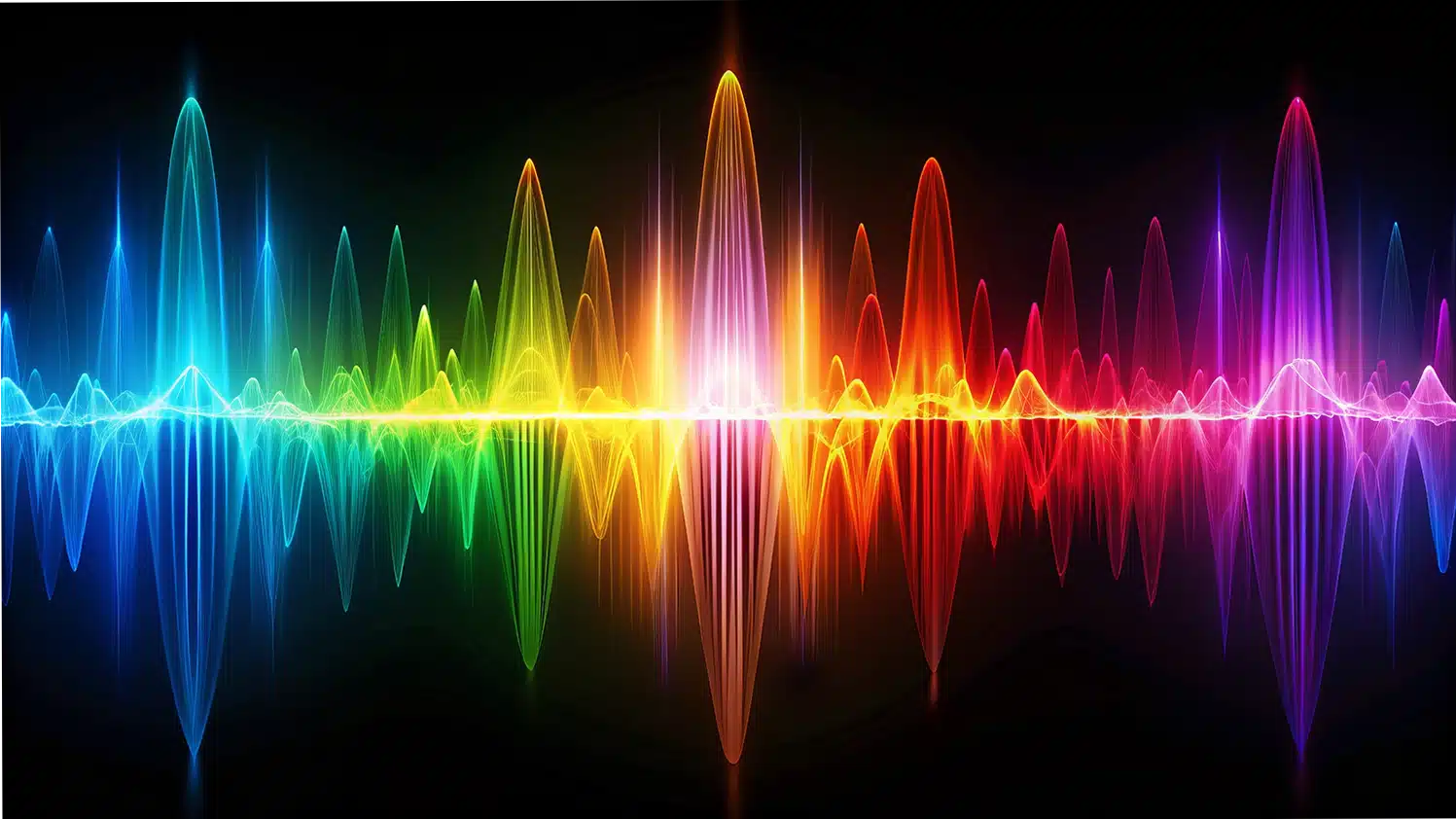
Sound.
As music, it can evoke the feeling of a sentiment perfectly expressed. In the human and animal world, it is often the vehicle to provide comfort and social cohesion — or quickly communicate information needed for safety and survival. The ability to perceive sound is one of our key senses and its waves surround us, operating invisibly.
Sound, however, does so much more. Sound waves produce vibrations in the particles of a medium like air, water or a solid object. Depending on the medium, sound waves with enough intensity and frequency can pass through objects and even manipulate matter. How they moves through matter can also provide data on objects we can’t directly see or touch.
Ultrasound technology is an example of this kind of acoustic energy created using high-frequency sound waves of 20 kilohertz or greater. As the wave frequency increases, so does the pitch, resulting in the fact that ultrasound waves are audible to some people but not all.
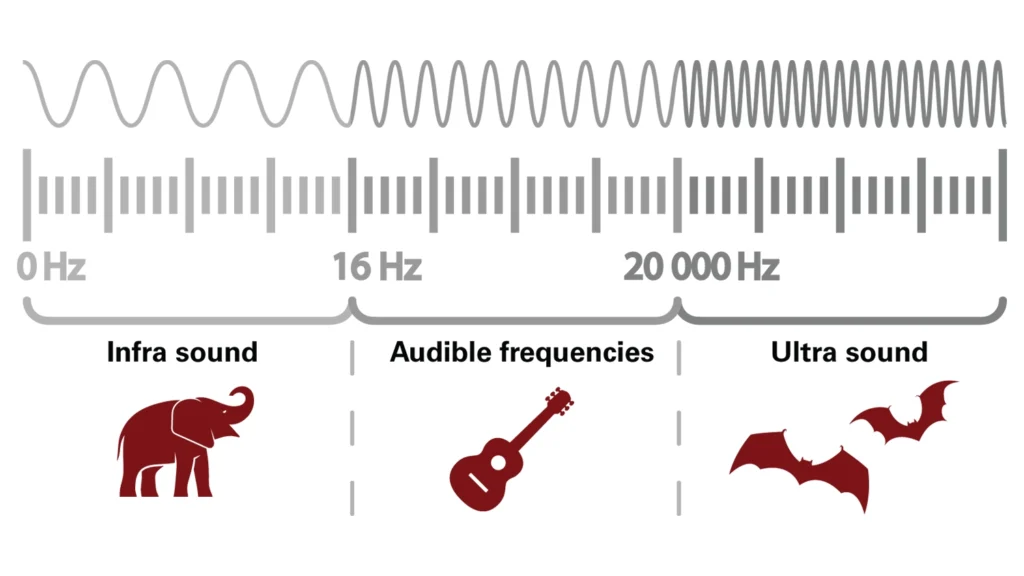
Most people are familiar with ultrasound’s conventional usage as an imaging modality used during pregnancy. An ultrasound machine uses sound waves to create images of the inside of the human body. To do this, a device called a transducer probe sends out high-frequency sound waves that bounce off of fluids, tissues and organs. Similar to the physics of echolocation, the amount of time it takes for the probe to emit a signal and receive the rebounding sound waves conveys data on the dimensions and size of, for example, an unborn baby.
Researchers at NC State University are taking ultrasound’s potential to the next level in medical applications. One study has found that measuring ultrasonic vibrations can provide accurate diagnoses of lung disease.
In another, a device that creates a pulse of sonic waves in an intravenous catheter can create enough shear force to gently unblock dangerous blood clots in the venous sinuses, a part of the brain’s blood drainage system connected to the heart.
In a third study, ultrasonic waves help better organize cells in 3D-printed tissues to mimic the function and biological structure of organic tissues.
Continue reading to learn more about how these NC State researchers are studying cutting-edge ways for medical professionals to leverage the power of ultrasound.
Measuring Vibrations to Diagnose Lung Disease
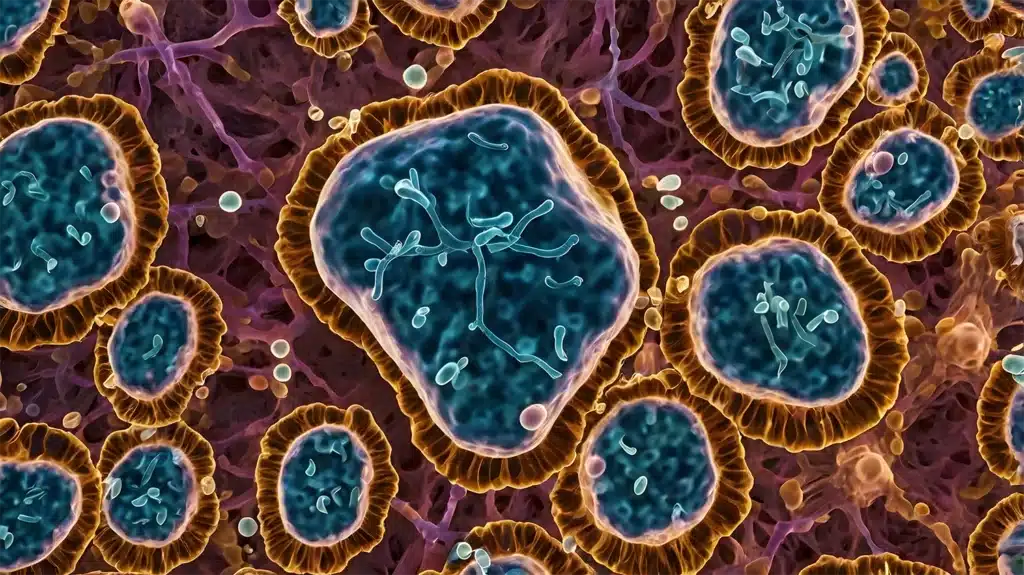
At the College of Engineering, NC State researchers have established a set of quantitative parameters for using ultrasound to diagnose chronic lung diseases.
Marie Muller, an associate professor of mechanical and aerospace engineering at NC State, has been leading the research team in defining quantitative ultrasound (QUS) parameters — or numeric measurements — for lung health.
Dr. Thomas Egan, a surgeon in NC State and UNC-Chapel Hill’s Joint Department of Biomedical Engineering, and Jonathan Mamou, a researcher in Weill Cornell Medicine’s radiology department, jointly supervised the research with Muller — and together, they defined diagnostic parameters that can distinguish one type of lung disease from another, proving the accuracy of their technique in animal models.
Diseases can alter the structures and other characteristics of the lungs. QUS can detect these changes by measuring how high-frequency sound waves vibrate through or bounce off internal structures of the lung.
Diagnosing lung disease with traditional imaging ultrasound can be challenging. Typically, a medical technician examines a grainy image and makes a diagnosis based on certain visual features that appear in the lung. However, these features can indicate a range of conditions or illnesses — and lead to a misdiagnosis.
For example, pulmonary edema is a potentially dangerous condition where the alveoli — tiny air sacs responsible for delivering oxygen to the blood — become filled with fluid. With traditional ultrasound, edema can be challenging to diagnose because, in the grainy ultrasound image, it may look similar to pneumonia or other lung diseases.

QUS, on the other hand, produces data based on how the ultrasonic sound waves vibrate through or bounce off the alveoli. Instead of depending on a technician’s subjective interpretation of a grainy image, QUS produces a number that reflects a physical quantity or aspect of the lung’s tissue or structure.
In fact, where traditional ultrasound struggles, QUS excels. Muller’s research team explains that traditional ultrasound has difficulty visualizing the lungs due to the irregular surface of the alveoli’s clustered air sacs. Sound waves, instead of reflecting directly back to the probe, scatter as they bounce off the alveoli. This scattering makes it difficult to obtain a clear image.
QUS, however, leverages this scattering effect. The increased movement of the sound waves ping-ponging around the lungs’ internal structures produces a greater number of data points and more information. The speed of the sound waves’ movement around can reveal signs of edema in the alveoli, such as whether the air sacs are filled with fluid — and if so, how much.
“You can measure the severity [by] the density of alveoli,” Muller said. Since QUS is based on measuring the physical changes, it has the potential to be more accurate and objective than the process of interpreting the visual features in an ultrasound image.
A Sound Wave Vortex Safely Speeds Up Blood Clot Treatment
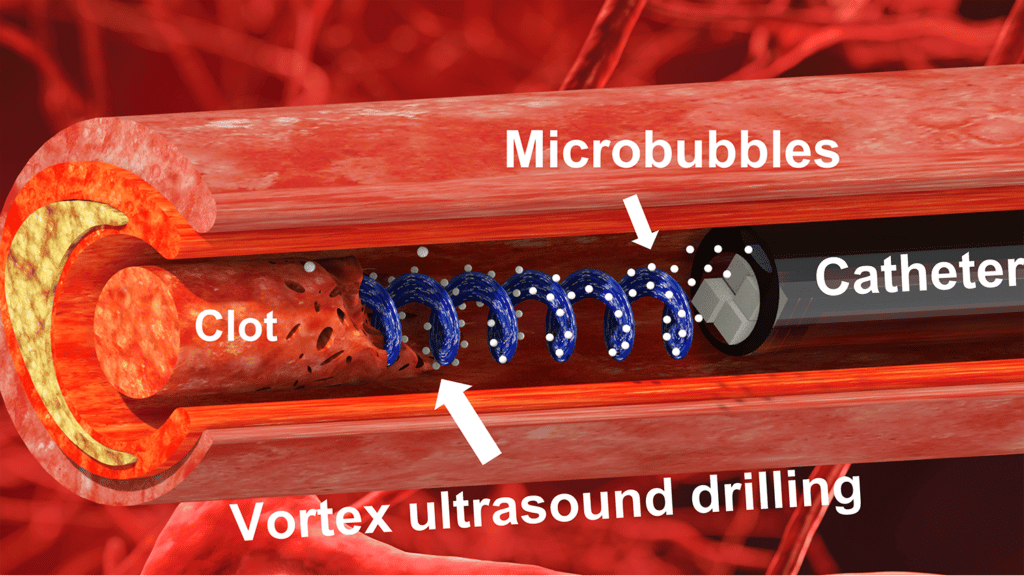
In another corner of the mechanical and aerospace engineering building, Xiaoning Jiang and his research team have been pioneering a novel intravenous treatment called “vortex ultrasound.” The technique innovates existing ultrasound technology to treat blood clots from Cerebral Venous Sinus Thrombosis (CVST). With vortex ultrasound, tornado-like waves can produce enough shear stress to gently break down blood clots in the brain faster and safer than existing methods.
Cerebral venous sinus thrombosis (CVST) occurs when a blood clot forms in the veins responsible for draining blood from the brain. It has become more prevalent since the outbreak of the coronavirus pandemic and has become a common cause of stroke in younger people. The current treatment for CVST fails in as many as 20-40% of the cases.
“Once the CVST blood clot forms, it blocks the brain venous blood flow. Doctors tell us they have a very short window to reopen it,” said Xiaoning Jiang, a distinguished professor of mechanical and aerospace engineering and a University Faculty Scholar at NC State. “Otherwise the risks can be significant brain damage or even life-threatening.”
In proof-of-concept in vitro testing, Jiang’s team demonstrated that their vortex ultrasound is effective in dislodging a CVST blood clot in less than half the time existing methods take.
The technique uses a transducer small enough to fit into a catheter that’s fed through the circulatory system to the site of the blood clot. High-frequency sound pulsations create a vortex — tiny swirling, tornado-like waves — in fluid, producing sufficient shear stress to gently dislodge the blood clot from CVST. Combined with microbubbles also fed into the catheter, the swirling waves can dislodge a blood clot in less than 10 minutes — a fraction of the time it takes for existing CVST treatments.
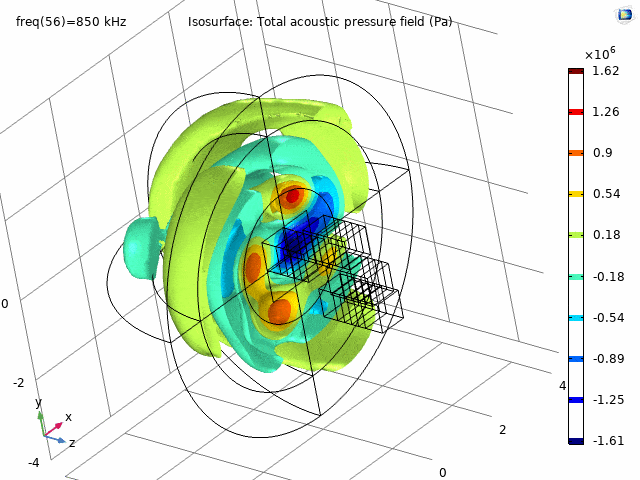
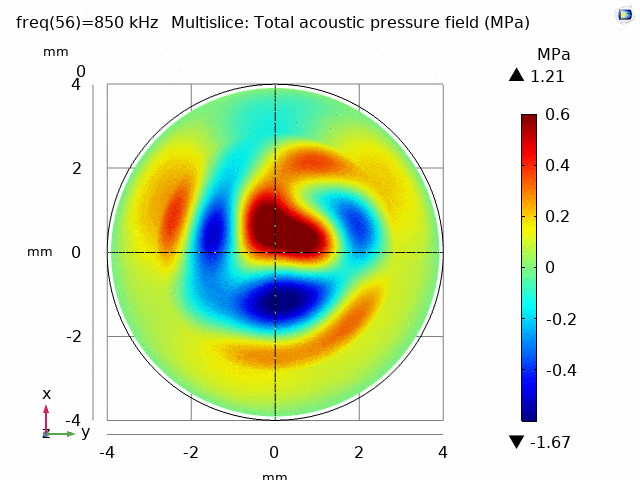
“Based on available data, pharmaceutical interventions to dissolve CVST blood clots take at least 15 hours, and average around 29 hours,” said Chengzhi Shi, co-corresponding author of the work and an assistant professor of mechanical engineering at Georgia Tech. “During in vitro testing, we were able to dissolve an acute blood clot in well under half an hour.”
The research team’s technique enhances sonothrombolysis, a procedure that uses traditional ultrasound and microbubbles to break up and remove blood clots. With traditional ultrasound, high-frequency sound waves produce pressure to exert force on particles or tissues in their path — but they cannot produce enough pressure to disturb a CVST blood clot.
The vortex effect, on the other hand, generates higher shear stress with helical-shaped waves that swirl as they move forward. These tornado-like waves are more effective and quicker at disrupting a blood clot than traditional ultrasound.
“Based on our in vitro testing, this approach eliminates blood clots more quickly than existing techniques, largely because of the shear stress induced by the vortex wave,” said Jiang, the Dean F. Duncan Professor of Mechanical and Aerospace Engineering at NC State.
Jiang’s team is currently experimenting with various transducer designs to optimize the vortex effect. Jiang said that they have successfully replicated similar results using a 3D-printed lens and are now developing multi-channel electronics to further refine the technique.
Achieving Biomimicry in 3D-printed Tissues

Elsewhere in the College of Engineering, researchers have developed a way to use ultrasound to improve 3D-printed tissue’s ability to mimic and function like natural, organic tissue.
Current technologies can create medical products like knee implants by printing living cells. However, one of bioprinting’s major challenges has been effectively organizing these cells so that the engineered tissue achieves biomimicry, or in other words, imitates organic tissue’s biological structure and functioning.
Rohan Shirwaiker, the James T. Ryan professor in NC State’s Edward P. Fitts Department of Industrial and Systems Engineering, is leading a team that aims to tackle that challenge. They’re innovating the bioprinting process by using ultrasound waves to organize the cellular structures in printed tissues so that they more closely resemble — in form and function — the real, organic thing.
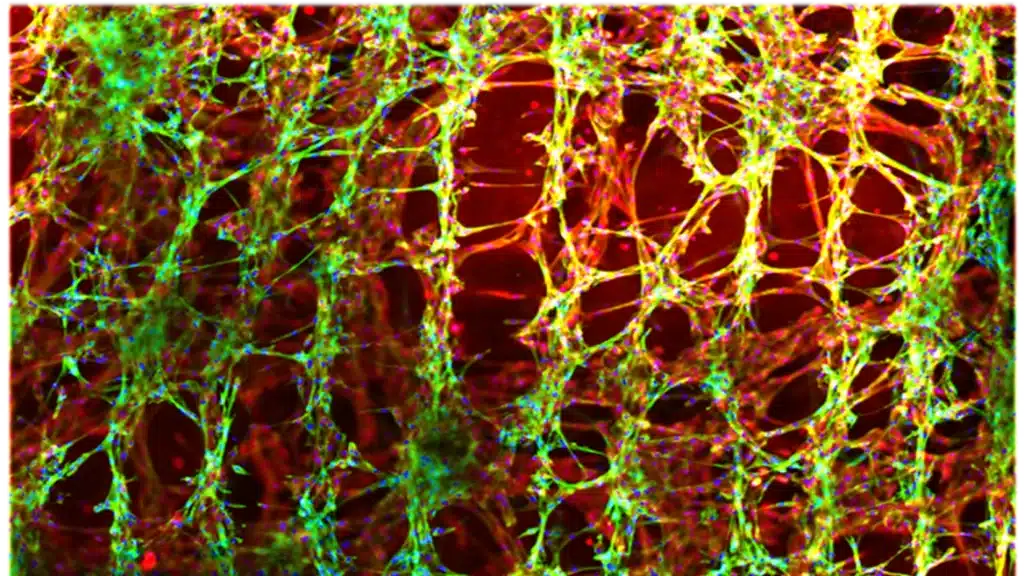

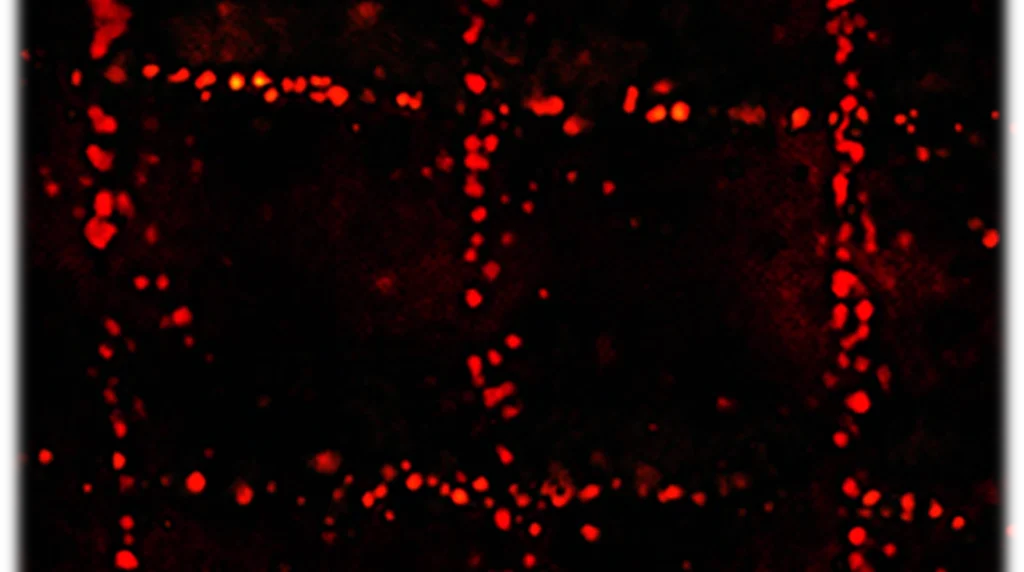
“Through this ultrasound-assisted biofabrication process, we are studying how in conjunction with 3D printing, we can use ultrasound waves to organize cells and collagen and other fibers to mimic the organization of natural tissues,” Shirwaiker said.
Cells, collagen and fibers naturally grow in certain patterns so they can carry out their biological functions. The research team is seeking ways to mimic these natural patterns in biofabricated tissues so that they can carry out the same functions.
“The ultrasound waves — the vibrations — are pushing the cells into patterns,” Shirwaiker said. “[The technology] is able to organize single cells and fibers into a pattern that we want to make tissues that are functional — mechanically and biologically — inside the body.”
To achieve the desired pattern, the researchers built specialized ultrasound chambers that direct ultrasonic waves across the area where living cells are printed. The soundwaves travel in one direction and then bounce back, guiding the cells into rows and aligning them at the points where the waves intersect with their reflections. The technique has been proven effective in organizing the cells into a 3D matrix that resembles a natural tissue’s structure.
Shirwaiker’s team has successfully printed a knee meniscus — one of the trickiest types of tissue to print — that closely mirrors its organic structure. Since the knee joint must bear significant weight, engineering artificial tissue accurately for it is especially crucial.
“The knee meniscus has a very unique organization and a biomechanical function in the body,” Shirwaiker said. “When we walk, run and perform other routine activities, that tissue has to absorb a lot of the load and support our ambulatory functions.”
“Our [ultrasound-assisted biofabrication] process is one of the very few that can create the three-dimensional organization in a very organically shaped new meniscus.”
Shirwaiker said that he and his team have a patent pending for their ultrasound-assisted process.
Safe and Affordable: Ultrasound Evolves
For Shirwaiker, Jiang and Muller, one of the biggest advantages of ultrasound is that it doesn’t cost much to manufacture. Looking toward the future, the affordability of ultrasound technology paired with machine learning and AI will push innovations even further.
Portable and wearable ultrasound devices that use machine learning are being created for the not-too-distant future. Such devices would support machine learning and could use the QUS parameters Muller’s team developed. These ultrasound-based health aids would be useful for chronic disease, allowing patients ongoing and immediate monitoring without requiring a trip to a medical facility.
“The time is ripe right now with the advances in the hardware,” Muller said. ”It’s amazing what people can do right now with stretchable, stickable, even implantable or swallowable ultrasound devices. Combine that with quantitative ultrasound and machine learning, and you have a completely integrated intelligence system that people can take home.”
Muller believes innovations in ultrasound coupled with advances in hardware have the potential to help millions of people.
“So many people don’t have access to healthcare, so it matters a lot to me,” Muller said. “I see it as an empowerment tool for people to take care of their own health.”
In the meantime, the research continues. It seems apt that Muller, Jiang, Shirwaiker and their research teams are leveraging how sound waves pass through objects and beyond boundaries. Likewise, the innovative potential of ultrasound and these dedicated scientists at NC State are limitless.