How Long Can Viruses Survive in a Dead Body?
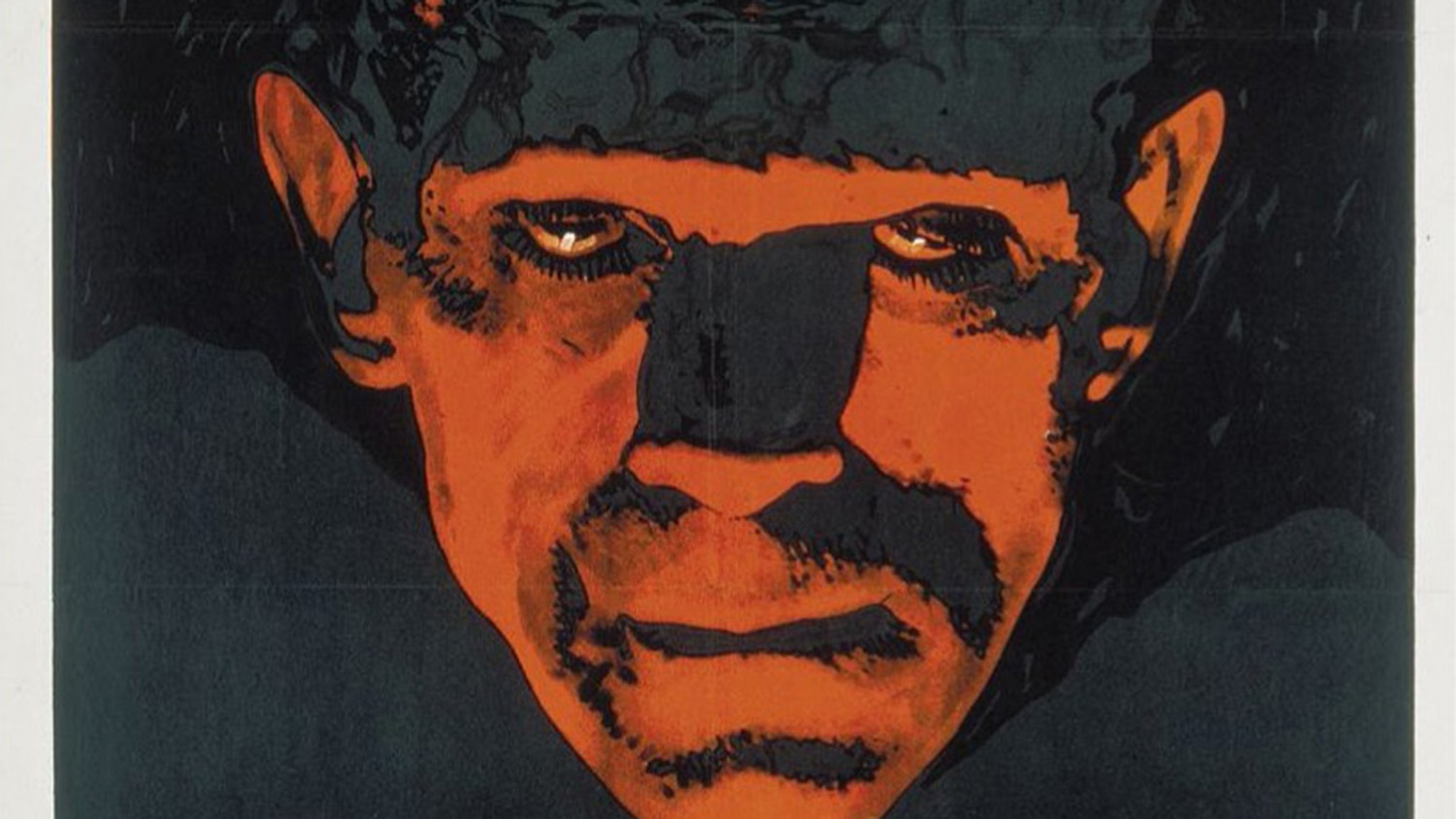
People have a lot of questions about viruses right now. Some of those questions involve what happens to viruses in the remains of humans or animals. For example, could people get sick from digging up decades-old bodies? Or could future scientists get more information about COVID-19 by examining human remains a century from now?
To address these questions, and many more, we spoke with Matt Koci, a virologist and immunologist whose work focuses on host-microbe interactions in birds. Koci is a professor in NC State’s Prestage Department of Poultry Science.
The Abstract: How long would viruses in a dead body pose a biological hazard? For example, would the disinterred remains of an animal or human pose a biological threat (because of a virus) after a week? A month? A year? A century? Does it vary from virus to virus?
Matt Koci: Unfortunately, like so much with diseases and how they spread, the answer depends on lots of factors. Probably the biggest factor is what kind of virus it is. What is its virion made of? Does it have a capsid or an envelope?
TA: Time out: what’s a virion? What’s the difference between a capsid and an envelope virion? And how does that affect how long a virus retains the potential to make someone sick?
Koci: Sorry. Virion is the $5 word for virus particle. In order for a virus to move its genome from victim cell to victim cell it needs protection from the environment. There are two main ways viruses do that. One way is for a virus to surround its genome with a lipid bilayer membrane (a two-layer shell made out of fat that it steals from the cell it’s hijacked). These viruses are called enveloped viruses, because they’re in those membrane “envelopes.” The second way a virus can protect itself from the environment is by building a little geodesic shell of proteins around its genome, forming what is essentially a little protein BB or golf ball. These shells are called capsids, so viruses that form these protective layers are called capsid viruses.
Because the virus particles of enveloped viruses are largely made up of lipids (fats) they tend to be less able to survive in the environment as compared to viruses with capsids. Enveloped viruses are more prone to drying out, and they are sensitive to a lot more disinfectants than capsid-based viruses. That’s why handwashing with simple soap is effective against viruses like influenza virus and coronaviruses (which are enveloped viruses), but soap won’t work for viruses like norovirus (which has a capsid shell).
Soap works because it has lipids in it too, and the lipids in the soap insert themselves into the virus envelope in a way that causes the envelope to fall apart – inactivating the virus. Soaps don’t work on capsid-based viruses because there’s no lipid in the virus particle for the soap to interact with. That’s why we need other types of cleaners, like bleach, to tackle non-enveloped viruses.
TA: OK, going back to the question of how long viruses remain hazardous: is envelope versus capsid the only issue?
Koci: I think what the virus is made of is probably the biggest issue affecting how long it could stick around and still be a hazard to others. But it’s not the only factor. The temperature and humidity of where the body is, where the virus is located in the body, how much virus is there when the person died, the burial process, and how deep the body is buried would all influence how long virus associated with that body might pose a risk.
The good news for us is that unlike bacteria that can grow on their own, viruses have to be inside living cells to replicate. So when the body dies the virus can’t replicate anymore; it’s just a question of how long will it take for all the virus that is there to no longer be infectious. But this isn’t like the end of the first Avengers movie. You don’t flip a switch and instantly all the faceless alien invaders fall over dead. You have to go around and snuff out each one. How long that takes depends on how big the alien horde is, where they are and how much body armor they have. However, in this analogy, temperature, humidity and enzymatic juices stand in for Avengers.
TA: Do viruses do better when it’s hot or cold? Do they like wet environments or dry ones?
Koci: For the most part the colder things are, the longer viruses will stay active (i.e. capable of infecting you); and the warmer things are, the faster they lose infectivity. When we work with viruses in the lab, unless we are infecting cells or animals (which would be at 37C/98.6 F) we try and keep things cold. For short-term storage (a few days) we’ll keep the virus in the refrigerator (4C/40F). For long-term storage (weeks, months, years) we’ll keep the virus in an ultra-cold freezer (-80C/-112F).
As for humid versus dry environments, for most viruses, especially enveloped-viruses, dry environments lead to faster rates of inactivation. In both cases, in general, non-enveloped viruses can tolerate higher temperatures and lower humidity levels than enveloped viruses, but it’s really the drying out part that is tough on enveloped viruses. How sensitive they are to temperature is related to how humid the environment is. However, humidity is so much harder to measure and control for, compared to temperature, so we have a lot more data about how viruses handle temperature and not a lot about humidity.
What About COVID-19?
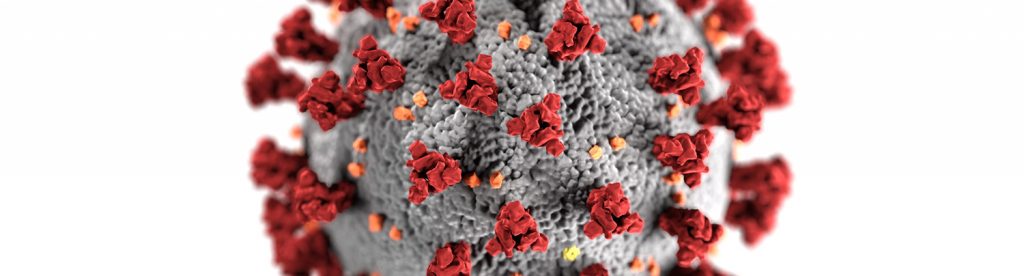
TA: You already mentioned that SARS-CoV-2 – the virus that causes COVID-19 – is an envelope virus. Do we know how long SARS-CoV-2 could remain viable in a dead body?
Koci: I have not seen any studies on this specifically. There are so few labs around the world with the type of building safeguards to work with this virus, and right now they are all focused on making vaccines or trying to find antiviral drugs that might work.
However, we can make some educated guesses based on what we know from other viruses. A lot of what we know about virus survival in bodies comes from food animals. The outbreaks of major diseases in poultry or livestock can cause massive economic damage, so a lot of research goes into managing these events. A big part of that research is making sure that we don’t spread the disease around by accident as we’re cleaning farms and removing carcasses.
In studies looking at how bird flu (avian influenza virus, an enveloped virus) survives in chicken carcasses, 90% of the virus was inactivated in around 15 days when the carcass was left at room temperature. But if the carcass was held at refrigerator temperatures (4C/40F), the virus lasted 4.5 times longer – that’s more than two months. That 15-day timeframe at room temperature was for tests looking for the virus in muscle. The 90% inactivation timeframe was around 10 days in feathers and was less than a day in the liver, at room temperature.
These different times in different tissues are to be expected. As the body breaks down after death, the cells of different tissues fall apart and the digestive enzymes inside those cells spill out. Since the liver’s job, among other things, is filtration and detoxification it has a lot more enzymes than other tissues. So when liver cells rupture, these enzymes can end up chewing up the virus. Feathers on the other hand are largely like hair. There aren’t cells to fall apart, and hence no enzymes released, but since they’re external they are more prone to drying out over time – and drying out is typically bad for enveloped viruses. Muscle, though, has relatively fewer enzymes than the liver but is still internal, which helps keep the virus at a constant temperature and humidity for a longer period of time.
It’s also important to remember that while 90% sounds like a lot, you have to keep in mind that viruses make a lot of copies of themselves. It’s not unusual for viruses to make billions if not trillions of copies. So, if it takes 15 days at room temperature for 90% of the virus to be inactivated and you have 1,000,000 viruses there, it could take as long as 105 days before all the virus is gone. In reality, it might not take all that long because inactivation isn’t perfectly linear over time, and as the body sits there microbes will start to decompose the body and produce heat which will speed things up, but you get the idea. It’s more than just a week or two.
Finding Evidence of a Virus
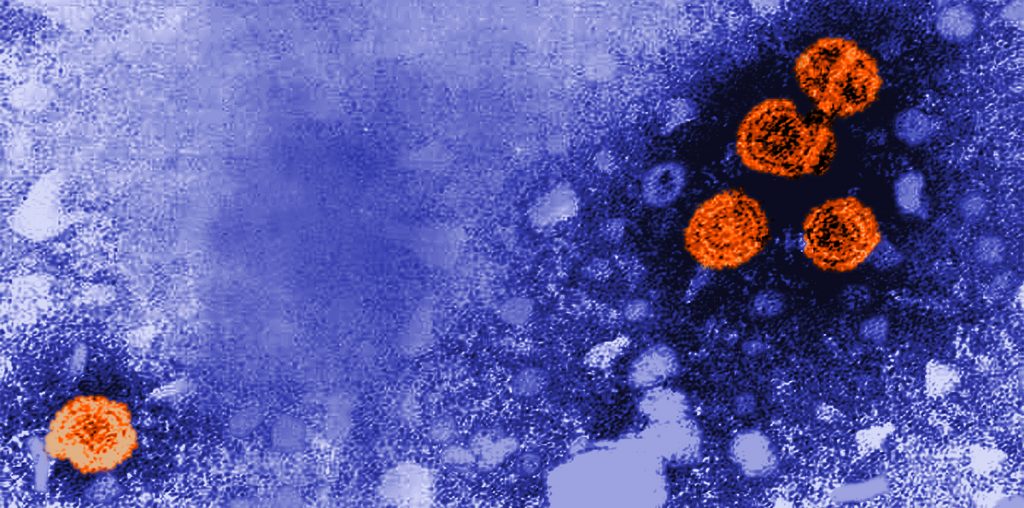
TA: How long would it be possible to detect a virus in a dead body, regardless of whether it poses a health risk? For example, would we be able to examine animal or human remains 10 years from now to better estimate the impact of COVID-19?
Koci: This is great question and an important distinction, because most of the data we have about SARS-Cov-2 survival is actually from a laboratory test called reverse-transcriptase polymerase chain reaction, or RT-PCR for short. This lab test detects the presence of the virus’s genome. Genomes will be there whether the virus can still infect you or not. This type of test is a lot more sensitive than the tests used to detect infectious virus. If you’ve been tested, or you’ve seen video of people getting the nasal swab with that 4-foot-long Q-tip, that swab collects samples for the RT-PCR test.
The RT-PCR test tells us if the virus is there. At the beginning of your infection and during the time you feel horrible, the assumption is (and it’s a really safe assumption) that if you’re RT-PCR positive you are infected and you are very likely to be contagious to others. At some point after you recover you are still RT-PCR positive for virus, but you might not actually be contagious anymore. That’s because some of the pieces of virus being detected may just be virus shrapnel left over from the war waged between your immune system and the virus.
We don’t have any SARS-Cov-2 data yet as it relates to dead bodies, but we can look at examples from other viruses again. Typically, if infectious virus is detected for days to weeks, the genome of the virus can be detected for months to years. One study looking at how long Ebola virus might survive after death could detect the infectious virus 10 days postmortem but still detected the virus genome 10 weeks later.
TA: Can we identify viruses in remains from decades or centuries ago, based on identifiable DNA or RNA (as opposed to making a diagnosis based on physical symptoms)?
Koci: So, the short answer here is yes. The oldest virus I know we’ve been able to actually detect was found in a 7,000 year old tooth from what is now central Germany. Researchers were able to detect and sequence the whole genome of the hepatitis B virus, or HBV, in this ancient tooth. HBV has a DNA genome instead of an RNA genome (coronavirus, influenza virus, and Ebola virus have RNA genomes).
So just a quick aside. Structurally, viruses come in two flavors: enveloped and capsid like we discussed above. The other major division among viruses is what their genome is made of: RNA or DNA. As a molecule, DNA is much more stable in the environment and can apparently survive in a tooth for thousands of years. It’s unlikely that we’d be able to detect RNA viruses that far back, but depending on what happened to the body of the person when they died, maybe.
If the person was embalmed, frozen or if tissues were collected at an autopsy we could use those to identify or even diagnose viruses. In fact, people have already done that.
The last great pandemic – the influenza pandemic of 1918 – came at a time before we even knew viruses were a thing. It wasn’t until the 1930s that we figured out what viruses were, but by then that particular virus was gone. Since we didn’t know what it was at the time, samples weren’t collected or stored in a way that made them of any use later. So, for decades we were left speculating what may have been so different about the 1918 virus from the strains of influenza that came after it. That was until the late 1990s, when Jeffery K. Taubenberger and a team at the Armed Forces Institute of Pathology decided to go through the tissue archive and find preserved tissue samples from soldiers who died from pneumonia-like symptoms during the pandemic. Taubenberger and his colleagues developed methods for extracting RNA from these tissue samples (something people didn’t think was possible at the time) and were able to pull out small bits and pieces of the influenza virus genome. Over several years, they were able to piece together the whole genome of the 1918 influenza virus – and they used that to resurrect this extinct virus.
Why You Resurrect Monsters
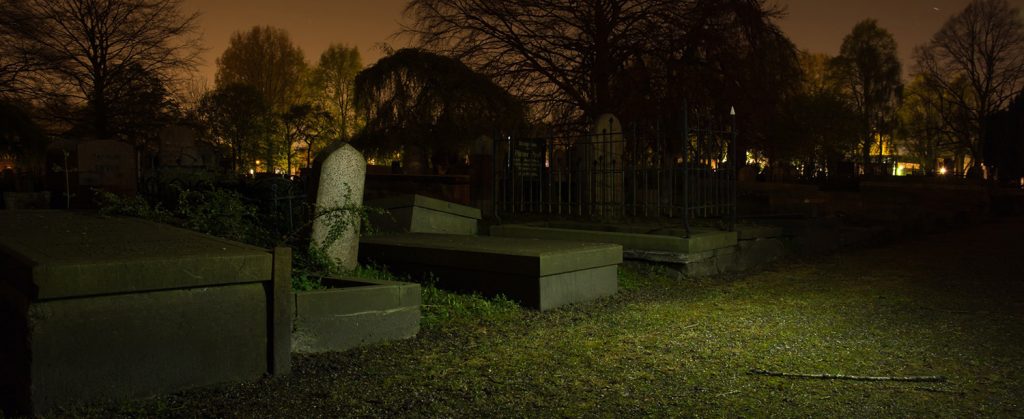
TA: Time out, again: why did Taubenberger want to resurrect the 1918 influenza?
Koci: Yeah, I know that’s most people’s reaction just before they start quoting Dr. Ian Malcolm from “Jurassic Park.” Until COVID-19 came along, most people had been expecting the next pandemic would be another influenza virus. Looking back through history, we’ve had an influenza pandemic about every 30 or so years. Everyone knows about the big one in 1918, and we’re pretty sure one happened in 1890, but we also had the Asian flu in 1957-58, and the Hong Kong flu in 1968-69. So in the late 1990s, most virologists were starting to brace for the next flu pandemic. We had surveillance systems and vaccines, so we assumed we were better prepared, but the 1918 pandemic was so much worse than any of the others since and we didn’t know why. There were lots of ideas about why it was worse, but no way to know if any of those ideas were right because we didn’t have that virus to study in the lab.
Then in 1997 the H5N1 avian flu emerged. And while it was devastating to poultry, it was also able to infect and kill people. We had never seen a flu virus jump straight from birds to people before. Could that have been how 1918 started? Could there be clues in the 1918 virus that would tell us what to look for in new strains of flu that would give us early warning of a new pandemic strain?
So the 1918 virus was resurrected to try and understand what made that pandemic worse than others in the hopes of helping us be better prepared for the next one.
TA: So, what did he learn? Was there something special about that virus?
Koci: This work took about eight years and a team comprised of at least three different institutions. And from all that time and effort, the biggest lesson we learned was that Mother Nature isn’t giving up her secrets that easy. The hope was that there would be some flashing red-light screaming “look at me!” to explain why the 1918 virus was so deadly. But the real answer is more complicated than that. There isn’t one series of events or changes that might give rise to a new pandemic strain; it’s the collection of several different changes coming together in just the right (or wrong) mix.
But we still learned a lot, and several of these lessons seem like ones we’re re-learning now. The virus came from birds, but it spent time evolving to infect humans in some other animal. What that intermediate host was or how long it took before it could infect people and spread from person to person we don’t know, but it was clearly different enough from other viruses that our immune systems weren’t prepared.
We learned that changes in the protein that the flu uses to get into our cells (HA protein) worked differently than most other influenza viruses, and if we moved just that gene into seasonal flu strains, they got more virulent. However, if we replaced the 1918 virus HA gene with the HA from the seasonal flu and left all the other 1918 genes the same, 1918 was just as virulent no matter which HA gene it had. So the HA gene plays a role but all the other genes do, too.
Ultimately, the lesson learned, or rather I think the lesson we were supposed to learn, was: don’t be so reactionary. Stop waiting for nature to spit out a new strain and then rush to make a vaccine to it. We learned we really need a universal influenza vaccine, a vaccine that will provide protection to all strains of the flu no matter what nature decides to throw at us. We also learned we need more weapons than just vaccines. We need more antivirals, designed to target all the different parts of viruses. And not just influenza virus but every virus we think might have pandemic potential.
We have made progress on these lessons. There still isn’t a universal flu vaccine on the market yet, but there are several in the latter stages of testing that sound promising. Also, we have a few more antiviral drugs today than we did 20 years ago, but clearly not enough to be ready for the next pandemic, this pandemic.
“Pandemic preparedness is a tough sell. When it works, nothing happens.”
TA: Is there anything else in particular you think is worth noting to a general audience from the broader field of research into historical viruses?
Koci: Nature gave us fair warning something was coming. H5N1, SARS, H1N1, and MERS were all warning shots. A lot of people have been ringing alarm bells for years, but pandemic preparedness is a tough sell. When it works, nothing happens and it looks like you wasted all that money.
The fact that H5N1, SARS and MERS never took off around the world was a mix of luck and great responsiveness by international, national and state agencies. H1N1 did take off around the world, but it turned out to not be as nasty as we feared. That was good luck, but it’s also worth noting that investments by the George W. Bush administration into federal agencies that support vaccine research (like BARDA) and rapid flu vaccines, and the vaccine plant here in Holly Springs had us an H1N1 vaccine in record time.
The lessons we’re learning today from COVID-19 build on the lessons we learned from the 1918 influenza. We aren’t the apex predator we think we are. An organism 750 times smaller than the width of human hair can wreak havoc on us to rival most wars, and every couple of generations nature releases one of these just to remind us who’s boss.
That said, at the end of the day, we’re going to beat this. The unprecedented, “all hands on deck,” collaborative approach by scientists around the world is bringing resources to this fight in ways that were unimaginable even seven or eight months ago. We will develop therapies and vaccines against this, and we’re going to do it faster than we ever have before. I just hope the lessons we all learn, not just scientists, stick with us for a while. If so, we’ll be that much better prepared the next time Mother Nature tries to bump us off.
- Categories: