Ultrasound and the Future of Medical Imaging
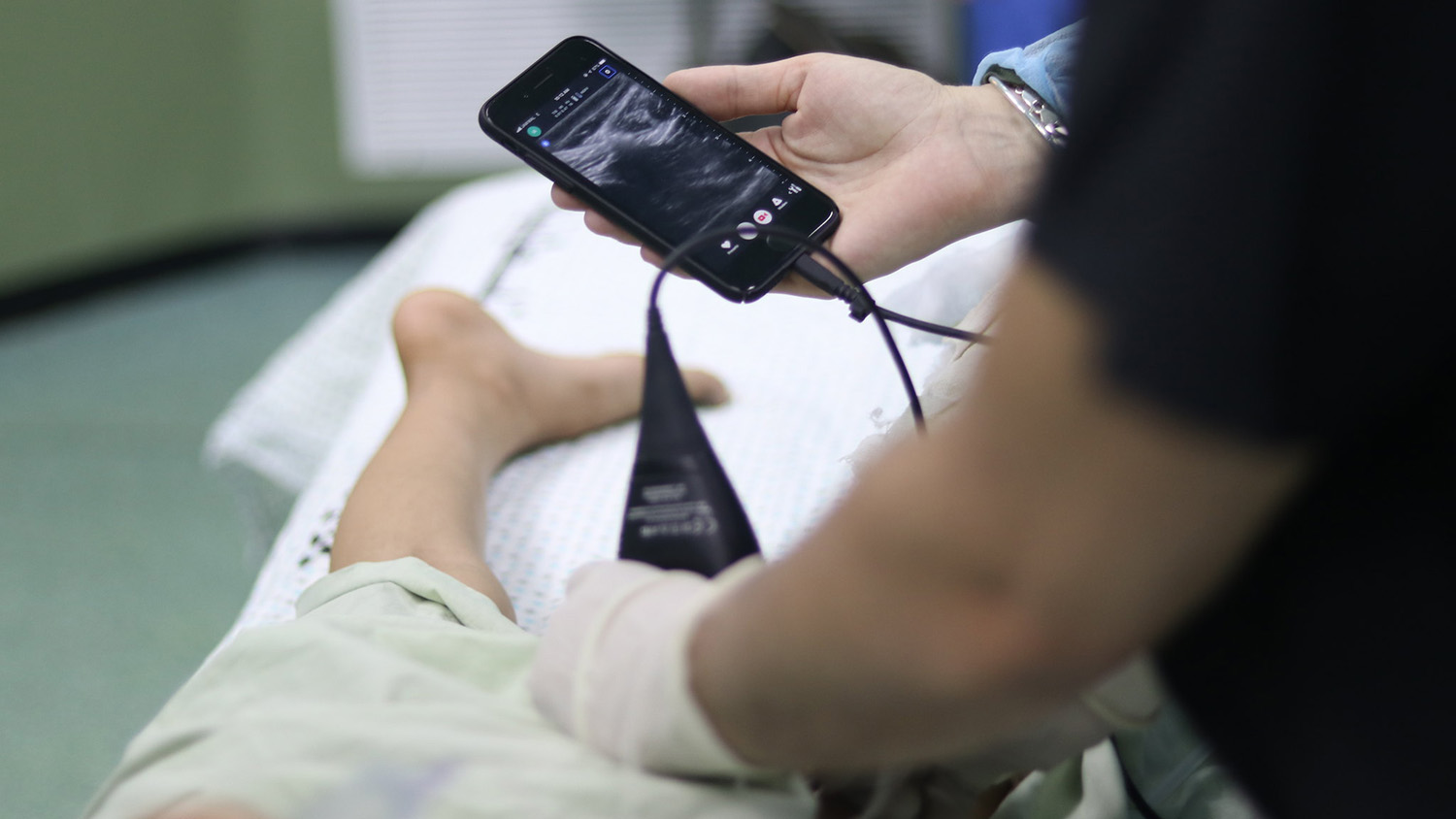
When most people think of ultrasound, they think of pregnant mothers and prenatal check-ups. But advances in technique and technology are making ultrasound increasingly valuable for imaging in a wide variety of medical applications, including pulmonary disease.
This is remarkable, in part, because it was once thought that ultrasound would never be useful in assessing lung health. And it is important because it can improve access to a valuable diagnostic tool.
To learn more about the new roles that ultrasound may soon be playing in clinical medicine, we spoke with Marie Muller, an expert in the physics of sound and an associate professor in NC State’s Department of Mechanical and Aerospace Engineering.
The Abstract: Threshold question: what is ultrasound and why do we use it in medicine?
Marie Muller: Ultrasound waves are high frequency sound waves. Their frequency can range anywhere between 20 kilohertz (kHz) and tens or hundreds of megahertz (MHz). Humans cannot hear sounds above 20 kHz, but dogs can. Bat signals can go up to 200 kHz.
Ultrasound is used in medicine as tool for creating images of structures inside the body. Ultrasound imaging is based on the principle of echolocation. An ultrasound pulse is sent into the body by an ultrasound probe. When the ultrasound pulse hits a structure inside the body, it is reflected to the probe. Because we know the speed of sound in the body, we just need to measure how long it took for the ultrasound pulse to be bounced back in order to estimate the distance of the object. This is how an image can be formed. Bats use the same principle to fly in the dark without hitting obstacles. They emit ultrasound pulses, wait to hear them back, and determine the distance. This is also how sonars work.
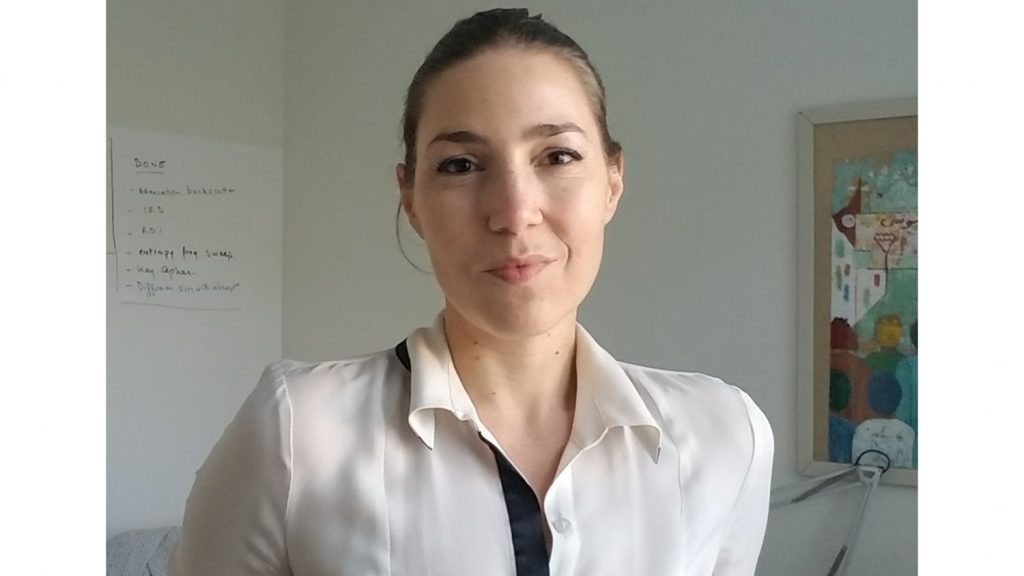
TA: Many people are familiar with the use of ultrasound to see what is happening in the human heart, or to observe developments over the course of a pregnancy. Why is it difficult to use ultrasound to observe what’s happening in the lungs?
Muller: The lung contains millions of air-filled alveoli. And those pockets of air scatter the ultrasound waves in random directions. Therefore, when an ultrasound pulse is transmitted into the lung and comes back to the probe, it is very difficult to know what path the ultrasound pulse has taken. The pulse probably traveled a long, random path in the tissue instead of propagating in straight line, as it would in other biological tissues. That makes imaging very difficult.
It’s like trying to identify the location of an object in a room where the walls would be paved with millions of pieces of broken mirrors, all facing random directions. You don’t know what is where, and you can’t get a clear picture.
TA: Why would you want to see what’s happening in the lungs?
Every innovation we make with ultrasound has the potential to affect millions of patients, including patients in rural areas and in developing countries.
Muller: Ultrasound is a great imaging technology for three main reasons. First, it is non-invasive and non-ionizing. This means that you can follow a patient’s progress over time and repeat the assessments multiple times without exposing them to dangerous radiation. This makes it an ideal tool for monitoring chronic diseases such as pulmonary fibrosis. It’s also great for monitoring a patient’s response to treatment over time. Second, it works in real time – there is virtually no time lag. For example, this means that one can observe dynamic phenomena and use ultrasound imaging to guide surgery. Third, it is relatively inexpensive and widely available. This is the aspect that interests me the most. Every innovation we make with ultrasound has the potential to affect millions of patients, including patients in rural areas and in developing countries.
TA: Why is pulmonary ultrasound getting more attention now? Is it because of advances in technology?
Muller: The COVID-19 pandemic has revealed an urgent need for a way to perform quick, easy assessments of the lung. Since thousands of people are presenting with COVID-related pneumonia, CT scanning is no longer an option.
Another important factor is that the first wave of the epidemic hit Italy especially hard, and ultrasound imaging was already more prevalent there. Therefore, we have seen a large number of studies presenting results of ultrasound imaging in COVID-19 patients. Unfortunately, the clinical scanners available in hospitals only allow conventional ultrasound imaging. They’re not designed for looking at the lung. As we said above, it’s difficult to use conventional ultrasound to get good images of the lung. Since people can’t really make images of the lung, they are looking for artifacts – which are phenomena that appear in ultrasound images that can be used to help diagnose specific conditions.
The COVID-19 pandemic has revealed an urgent need for a way to perform quick, easy assessments of the lung.
For example, if there is pneumonia, there will be areas of the lung filled with fluid. Ultrasound waves cannot travel straight through the packets of air in the lung, but those waves are able to travel through the parts of the lung filled with fluid. In other words, for critically ill patients, the room full of broken mirrors has areas without mirrors. This will show up on the ultrasound image as a vertical bright line, called a “B-line.” Recent studies have used the presence of B-lines to assess the severity of COVID-19.
Unfortunately, there is a big limitation to this approach: it is qualitative and not quantitative. It will tell you that a patient is not doing well, but it won’t say how bad things are. It is also not specific: the artifacts have the same appearance whether the patient has COVID, congestive heart failure, or lung fibrosis. Finally, we know that this approach is highly operator dependent. Depending on who is holding the probe and how, those B-lines may or may not appear.
This is what we are working on in my lab: how can we make lung ultrasound quantitative, specific and operator-independent? To do that, we leverage our knowledge of wave physics.
TA: Your work involves both “point-of-care” and surgical applications. I know point-of-care applications are things that you might see at your family physician, as opposed to in a hospital. Can you give me an example of how your work fits into a point-of-care setting?
Muller: Point-of-care medicine means medicine that is practiced with the patient, as opposed to imaging technologies such as CT and MRI scans, for which images are interpreted by a highly trained expert who is away from the patient. Point-of-care means a lot to me because I know it is where I can make a big difference for a large number of patients.
If you think about it, developing methods that are quantitative and operator independent means that any clinician, any technician, anyone in fact, can do the measurement. You don’t need a highly skilled, highly trained doctor to do it. In fact, with the turn ultrasound technology is taking, I can foresee how in the near future, you will be able to have a small probe at home, connected to your smartphone, that you can use to monitor your own health, like when you’re taking your temperature. But for that to happen, the technology has to be robust enough that it will give you an unambiguous number, not an image you need to interpret. This is one of the projects I am working on.
With the turn ultrasound technology is taking, I can foresee how in the near future, you will be able to have a small probe at home, connected to your smartphone, that you can use to monitor your own health.
In my lab, we develop specific ultrasound algorithms to detect and quantify pulmonary fibrosis and pulmonary edema. We rely on the physics of wave propagation in complex media to extract microstructural properties of the lung. Both fibrosis and edema change the microstructure of the lung. This in turn changes the way ultrasound waves interact with the microstructure, and we measure those changes. Pulmonary fibrosis and pulmonary edema (associated with congestive heart failure) affect millions. We aim at developing technologies that can be both specific and quantitative. This will allow them to be deployed widely and to be used often in private practices, for screening, diagnosis and monitoring. Monitoring is especially important in the context of pulmonary fibrosis, because new treatments have emerged in the past few years. We will want to follow these patients to see how they respond to these new treatments. [Editor’s note: you can learn more about Muller’s work on pulmonary fibrosis and edema here.]
TA: What about how your work might be used in a surgical context?
Muller: This is another aspect of our work. We have developed methods for the real time localization of pulmonary nodules, based again on the physics of wave propagation in complex media. Lung nodules are small masses of tissue in the lung, which can be benign or cancerous.
Nodules are detected by CT scanning. If they are peripheral enough, they are removed using Video Assisted Thoracic Surgery (VATS). Surgeons use surgical staplers to cut out the lesion, and it is critical that – if cancerous – no part of the tumor be left behind. This is not easy to do, because the surgeon can’t see the tumor during the surgery.
Surgeons sometimes try to palpate the nodule with their finger, but bad surprises are common. Either surgeons can’t find the tumor, or they do find it but are unable to remove it with safe margins. This is why a real time imaging modality that could be used during surgery is needed. CT imaging is great at detecting lesions, but it does not work in real time. The technology we have developed relies on the principle that lung tissue is a highly complex medium from the point of view of ultrasound waves, but that the nodule is not. By mapping the complexity of the tissue, we obtain a map of the tissue, and that – in turn – allows us to accurately detect and localize the nodule.
TA: What are some of the biggest challenges facing the field of pulmonary ultrasound right now? And how is the field addressing those challenges?
Muller: The biggest challenge in lung ultrasound stems from the fact that it is not currently quantitative, and not based on first principles.
Current methods in lung ultrasound are based on the same assumption as for other tissues: the assumption of a fairly homogeneous medium. Because the lung is so complex, these assumptions don’t hold. Consequently, lung ultrasound images are not an anatomical representation of the lung. This makes the uniformization of the processes very challenging, and makes quantification of lung damage impossible. Interpreting lung ultrasound is currently a highly subjective business.
We are working on improving this, with a group of lung ultrasound experts worldwide. We have developed a series of statements related to the topic, which will be submitted to a much larger community of experts. The statements will be graded using a Delphi method in order to reach a consensus. But it is also critical that this new consensus not be taken at face value, and that the community keep an open mind as research progresses and new tools become available.